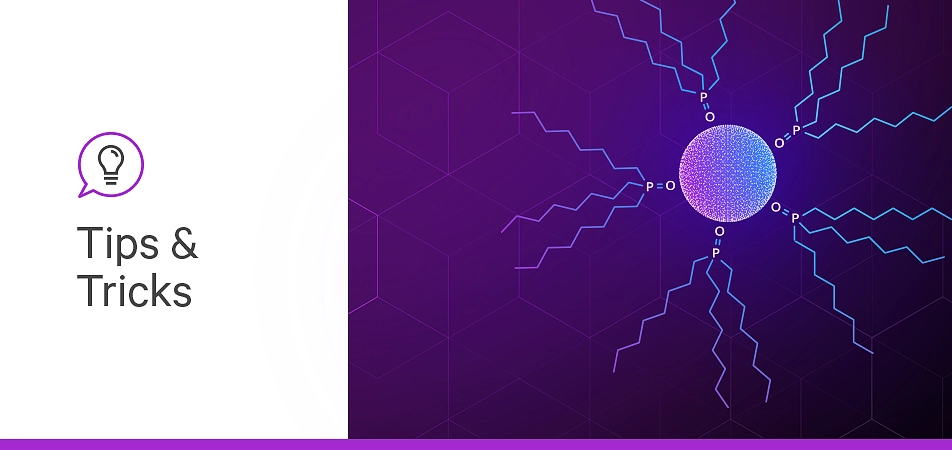
Bioconjugate therapeutics are among the most promising new biopharmaceutical modalities and are receiving increased interest from biopharmaceutical and research organizations, with antibody drug conjugates (ADCs) leading the way in terms of active development and progress to the clinic. Despite the immense potential of ADCs, the off-target toxicity associated with early ADCs delayed the class’s expansion. In first-generation ADCs, researchers had yet to explore how linker chemistry and bioconjugation strategy impact the controlled delivery of drug payloads. Now, it is well understood that careful linker design is essential for effective tumor targeting properties without affecting the non-tumorous entity. Recent advancements in linker technologies and conjugation approaches reignited interest in ADC biopharmaceuticals and have been driving development ever since.
Vector Laboratories’ BioDesign™ offers an extensive catalog of crosslinkers, including many with maleimide functional groups on one or more ends of linear and multi-armed discrete PEG (dPEG®) products. These crosslinkers enable the conjugation of thiols with amines or thiols with other thiols. The maleimide functional group reacts selectively with free thiols below pH 7.5. At pH 7.5 and above, the maleimide double bond reacts competitively with free amines (for example, lysine side chains) and free thiols.
Selecting the optimal maleimide crosslinker does not need to be an arduous task. In this guide, we will discuss amine-reactive, homofunctional, and other types of maleimide crosslinkers, and provide details to assist in the selection process.
At Vector Laboratories, we offer single molecular weight maleimide-functionalized dPEG® acids with lengths of 2, 4, 6, 8, 12, and 24 EO units. The terminal propionic group can be functionalized to form a variety of active esters. Alternatively, direct coupling of the acid to an amine is possible using DCC or EDC (often sold as the hydrochloride salt). Leaving the acid end unmodified after conjugating the maleimide moiety alters the conjugate’s surface charge. Surface charge modification may beneficially affect some drug or payload delivery applications.
Explore our MAL-dPEG®-acid crosslinkers:
N-Hydroxysuccinimide (NHS) esters are among the most common reactive acylating agents presently used in bioconjugation.[1] NHS esters were initially developed as homobifunctional crosslinkers.[2],[3] Today, they are among the most widely used amine-reactive crosslinking agents in bioconjugation.1
BioDesign MAL-dPEG®-NHS esters provide quality and consistent performance in research and manufacturing applications. We offer our products with linker lengths of 2, 4, 6, 8, 12, and 24 ethylene oxide units. Please see Figure 2 below.
Figure 2: General structure of MAL-dPEG®n-NHS ester crosslinkers.
Active esters based on 2,3,5,6-tetrafluorophenol (TFP; see Figure 3) are an increasingly popular alternative to NHS esters.
Figure 3: 2,3,5,6-Tetrafluorophenol is used to make MAL-dPEG®n-TFP ester crosslinkers.
TFP esters are more stable to hydrolysis in aqueous media than NHS esters, as discussed below in more detail. Shorter MAL-dPEG®-TFP esters may have slightly reduced water solubility due to the hydrophobic TFP moiety on one end of the crosslinker. However, using a dry water-miscible solvent such as DMAC or acetonitrile can overcome any reduced water solubility issue.
Figure 4: General structure of MAL-dPEG®n-TFP ester maleimide crosslinker products.
Figure 4 shows the general structure of seven of our eight MAL-dPEG®-TFP esters, while Figure 5 shows the specific structure of one unique product, MAL-dPEG®24-amido-dPEG®24-TFP ester. We designed PN11303 to provide a very long linker (158 atoms, 183.5 Å) between a thiol and an amine.
Figure 5: Structure of PN11303, MAL-dPEG®24-amido-dPEG®24-TFP ester
One possible application for PN11303 exists in MAL-dPEG®24-amido-dPEG®24-DSPE (Figure 6), which is PN11303 conjugated to 1,2-Distearoylphosphatidylethanolamine (DSPE) for use in lipids and micelles. The long discrete PEG linker allows for the conjugation of a payload such as a small molecule drug, targeting peptide, antibody, antibody fragment, or other protein. Moreover, the unconjugated maleimide moiety at the surface of a liposome may promote thiol-mediated cell-membrane trafficking.[4],[5] Other applications are certainly possible, limited only by imagination and nature’s laws.
Figure 6: Structure of PN11093, MAL-dPEG®24-amido-dPEG®24-DSPE. This product exemplifies an application of PN11303, discussed above. Please see the text for details.
TFP-functionalized MAL-dPEG® crosslinker products:
PN10553, MAL-dPEG®12-TFP ester
PN10554, MAL-dPEG®24-TFP ester
Selecting between a TFP-, NHS-, or acid-terminated MAL-dPEG® crosslinker requires a few considerations. Some customers choose crosslinkers based on their familiarity with the chemistry. NHS esters are the most common and most popular amine-reactive groups for reactive acylating agents. Thus, the chemistry is quite well-known, and NHS esters are selected based primarily on that awareness. While familiarity is reassuring, we strongly encourage customers to consider other factors as well.
One important factor to consider is whether you want to use an NHS or TFP ester. For example, a MAL-dPEG® acid should be used if the goal is to couple the MAL-dPEG®-acid via 1-hydroxybenzotriazole or another amine-reactive agent. Also, a carbodiimide can be used to couple a MAL-dPEG® acid directly to an amine without the need to form an active ester. If the direct coupling of the acid to an amine is desired, the acid-functionalized crosslinker should be used.
In 2017, Jian Wang et al. confirmed experimentally that TFP esters were superior to NHS esters for PEGylation.[6] The half-lives of both NHS and TFP esters decrease as the pH increases. However, TFP esters consistently display longer half-lives than NHS esters across a wide range of pH values. In aqueous media, the optimum pH for conjugating TFP esters to amines is higher (>7.5) than NHS esters (7.0-7.2).
For reactions in water or aqueous buffers, pH is another consideration for the maleimide group. At or above pH 7.5, the maleimide group reacts competitively with free amines, such as lysine side chains.1 However, the maleimide-thiol reaction proceeds optimally at pH 6.5 – 7.5,1,[7] while the reaction between TFP esters and free amines is very slow below pH 7.0.[8] In our experience, NHS esters should be used at pH 7.0 if the ester must be reacted before the maleimide. Conversely, if the maleimide can be reacted first, it may be possible to use a TFP ester provided that the maleimide-thiol reaction is conducted at pH < 7 to prevent unwanted TFP ester hydrolysis or acylation of amines by the TFP ester.
The water solubility of TFP esters should also be considered. Our shortest MAL-dPEG®-TFP ester products are less soluble in water than the corresponding MAL-dPEG®-NHS esters. The use of water-miscible organic solvents such as DMAC or acetonitrile may permit product dissolution, allowing the reaction to proceed; however, in cases where organic solvents are impermissible, NHS esters may be preferred over TFP esters. Water solubility is generally not an issue for TFP esters with dPEG®8 and longer linker chains.
The choice of solvent for the reaction is critical to success. In addition to choosing the correct pH for aqueous reactions, users should ensure that aqueous buffers contain neither free amines nor free thiols that can react with the crosslinkers. We recommend using dry solvents for reactions run entirely in organic solvents (drying for >48 hours over activated 3 Å molecular sieves is sufficient) free of amines and thiols. If an organic base is required to drive the reaction of the active ester with free amines, we recommend a highly hindered base such as 2,6-lutidine over more commonly used organic bases such as triethylamine.
4-formyl-benzamido-dPEG®12-EDA-MAL: a maleimide crosslinker that can form a Schiff base, secondary amine, hydrazone, or oxime bond
4-formyl-benzamido-dPEG®12-EDA-MAL, product number QBD-10075 (Figure 7), is another unique product in the BioDesign catalog. Like our MAL-dPEG®-acid and MAL-dPEG®-NHS/TFP ester products, PN10075 crosslinks amines and thiols. The bond formed between an aldehyde and an amine imine is known as a Schiff base. The imine reduces to a secondary amine with various reducing agents.
Figure 7: Full-length (top) and abbreviated (bottom) structure of PN10075, 4-formyl-benzamido-dPEG®12-EDA-MAL. This product reacts with amines to form imines (Schiff bases), hydrazides to form hydrazones, and aminooxy compounds to form oxime bonds. Please see the text for details.
Imine chemistry is dynamic, and that dynamism has proven highly valuable to synthetic chemists constructing supramolecular materials and stable exotic materials via dynamic covalent chemistry. A 2012 tutorial review by Matthew E. Belowich and Sir Fraser Stoddart provides excellent, clear details on dynamic imine chemistry.[9]
If the aldehyde end of PN10075 reacts with a hydrazide, it forms a hydrazone, which is stable in water but reverts to starting materials in the presence of mild aqueous acid. Hydrazone bonds are useful for forming prodrugs and other constructs that should degrade in acidic environments. If PN10075 reacts with an oxyamine, the bond formed is an oxime, which is more stable than a hydrazone bond.
Figure 8 shows the reaction between an amine and PN10075. The imine-forming reaction proceeds most quickly at pH 3 – 5 but can proceed at higher pH values with the aid of a catalyst.[10],[11],[12] High pH imine-forming reactions may necessitate reacting the maleimide end of PN10075 before the aldehyde end to avoid reactions between the maleimide and free amines. However, low pH reactions may not necessarily require that the maleimide-thiol conjugation occurs before the imine-forming reaction.
Figure 8: The reaction of PN10075, 4-formyl-benzamido-dPEG®12-MAL with an amine to form an imine (Schiff base).
Imines hydrolyze readily to starting materials in the presence of water. If aqueous stability is required, then the imine must be reduced to a secondary amine. Many agents reduce Schiff bases to secondary amines, including H2, borane, borohydrides, and silanes.[13],[14] Reductive amination of imines to secondary amines commonly uses sodium borohydride.[15] However, sodium borohydride also reduces aldehydes and ketones that may be present in the molecule. Milder reducing agents such as sodium cyanoborohydride[16],[17] or sodium triacetoxyborohydride[18] may be required. Water and protic solvents rapidly destroy sodium triacetoxyborohydride,[19] which may necessitate changes in the reaction setup.
Figure 9 shows an example reduction of an imine-containing conjugate of PN10075. In the example, the maleimide group is conjugated to a thiol.
Figure 9: Reduction of an imine (Schiff base) to a secondary amine with sodium cyanoborohydride.
Vector Laboratories offers a variety of BioDesign crosslinkers where all the terminal ends contain maleimide functional groups. We offer two homobifunctional maleimide products (two maleimide ends on a linear, discrete PEG), three homotetrafunctional maleimide products (four-armed discrete PEG), and one homohexafunctional maleimide product (six-armed discrete PEG). We build these products using our discrete PEG products. Two of the three homotetrafunctional maleimide products contain a pentaerythritol core, and one contains a tris core (Figure 10). The homohexafunctional maleimide product, PN11436, Hexa(-dPEG®11-MAL)dipentaerythritol, is built on a dipentaerythritol core (Figure 11).
Figure 11: Structure of Vector Laboratories’ crosslinker, PN11436, Hexa(-amido-dPEG®11-MAL)dipentaerythritol.
Homobifunctional Maleimide Crosslinkers
Homotetrafunctional Maleimide Crosslinkers
PN11433, MAL-dPEG®12-Tris(-dPEG®11-MAL)3 (Tris core)
PN11435, Tetra(-amido-dPEG®11-MAL)pentaerythritol (pentaerythritol core)
PN11414, Tetra(-amido-dPEG®23-MAL)pentaerythritol (pentaerythritol core)
Vector Laboratories offers two unique PEG-based maleimide crosslinkers built around a lysine core (Figure 12). bis-MAL-Lysine-dPEG®4-acid (Figure 12a), and bis-MAL-Lysine-dPEG®4-TFP ester (Figure 12b), both contain a dPEG®4 linker conjugated to the acid terminus of lysine and maleimidopropyl groups conjugated to each amine group of lysine. PN10630 has a propionic acid reactive terminus, while PN10631 is functionalized as a TFP ester.
Figure 12: Chemical structures of Bis-MAL-Lysine crosslinker products. a. PN10630, Bis-MAL-Lysine-dPEG®4-acid; b. PN10631, Bis-MAL-Lysine-dPEG®4-TFP ester.
The bis-maleimido groups can bridge disulfide bonds or, as shown by Tae Hyung Kim, Hai Hua Jiang, Seulki Lee, et al., conjugate two mono-sulfhydryl Exendin-4 peptides to one discrete PEG linker.[20] Moreover, PN10630 forms an essential part of two of our PK/BD modifier products, Bis-MAL-Lysine-dPEG®4-dPEG®12-Tris(-dPEG®24-acid)3, and Bis-MAL-Lysine-dPEG®4-dPEG®12-Tris(m-dPEG®24)3.
Vector Laboratories also offers a few maleimide crosslinker products containing no discrete PEG linker. These compounds can be building blocks for PEGylated crosslinkers or standalone crosslinkers for specific applications.
Figure 13 shows one example of a non-PEGylated maleimide crosslinker, Bis-Maleimide Amine, TFA salt, product number QBD-10232. This product contains bis-maleimide-functionalized lysine, similar to the PEGylated crosslinkers discussed above. However, 1,6-diaminohexane replaces the dPEG®4 linker of PN10630 and PN10631 as the linker arm in this product. PN10232 is quite hydrophobic and offers none of the benefits of our maleimide crosslinker products functionalized with discrete PEG.
Figure 13: Structure of PN10232, Bis-Maleimide Amine, TFA salt, a non-PEGylated maleimide crosslinker product.
A 2012 study by Scheer, Sandoval, Elliott, et al. successfully used PN10232 to design trastuzumab variants with customized F(ab’)2 domains. In this study, the authors introduced single cysteine substitutions into the VH and VL regions of trastuzumab’s Fab region. PN10232 then crosslinked different Fab units, creating custom-made F(ab’)2 units with new functions.[21]
Figure 14: MPS-acid, product number 10323, also known as 3-maleimidopropionic acid.
For customers who want to build their own maleimide-functionalized products or use maleimide alone as a very short crosslinker, Vector Laboratories offers 3-maleimidopropionic acid, product number QBD-10323, which we call MPS-acid (Figure 14), and NHS-3-maleimidopropionate, product number 10217, which we call MPS (Figure 15).
Figure 15: PN10217, MPS, also known as NHS-3-maleimidopropionate.
Product numbers QBD-10323 and QBD-10217 both react with thiol at the maleimide olefin bond. With PN10323, functionalizing the terminal acid with an alkylating agent such as NHS, TFP, or HOBt permits cross-coupling to an amine. However, using a carbodiimide such as EDC permits direct coupling of the terminal acid to a free amine. PN10217 can be directly reacted with an amine using a hindered base such as 2,6-lutidine, to drive the reaction. PN10323 and PN10217 are useful as very short crosslinkers and building blocks for new, longer crosslinkers.
Figure 16: Product number 10177, MPS-EDA∙TFA.
Finally, we offer product number QBD-10177, MPS-EDA∙TFA (Figure 16), a short, non-PEGylated maleimide crosslinker product. This product crosslinks a sulfhydryl group with a carboxylic acid, or it can be used as a building block with discrete PEG to form a new crosslinker having a longer spacer between the maleimide group and the PEG linker.
Other Thiol Reactive Maleimide Crosslinker Products
PN10630, bis-MAL-Lysine-dPEG®4-acid
PN10631, bis-MAL-Lysine-dPEG®4-TFP ester
BioDesign, by Vector Laboratories, offers an extensive catalog of maleimide crosslinkers that enable the conjugation of thiols with amines or thiols with other thiols. Numerous product applications exist for these crosslinkers, including antibody-drug conjugates (ADCs), fragment-drug conjugates (FDCs), protein-complex proximity analysis, FRET applications, modification of thiol-functionalized surfaces, supramolecular materials construction, and many more.
The maleimide crosslinkers available in the BioDesign portfolio of products benefit from our dPEG® technology. Discrete PEG products improve the hydrophilicity of poorly water-soluble products and reduce the immunogenicity of potentially antigenic compounds. Because these products are single molecular weight products, the analysis of bioconjugates is simplified compared to traditional, non-uniform (i.e., polydispersed) PEG products.
For more than two decades, Vector Laboratories’ maleimide-dPEG® crosslinkers have been trusted by researchers and industry professionals for use in diagnostic and therapeutic applications. We invite you to consider our highly cited BioDesign products and discover the dPEG® difference!
References
[1] Hermanson, G. T. Chapter 3, The Reactions of Bioconjugation. In Bioconjugate Techniques; Academic Press: New York, NY, 2013; pp 229-258. Greg’s book is the industry-standard reference in the science and technology of bioconjugation, and we sell it on our website.
[2] Bragg, P. D.; Hou, C. Subunit Composition, Function, and Spatial Arrangement in the Ca2+– and Mg2+-Activated Adenosine Triphosphatases of Escherichia Coli and Salmonella Typhimurium. Archives of Biochemistry and Biophysics 1975, 167(1), 311-321. https://doi.org/10.1016/0003-9861(75)90467-1.
[3] Lomant, A. J.; Fairbanks, G. Chemical Probes of Extended Biological Structures: Synthesis and Properties of the Cleavable Protein Cross-Linking Reagent [35S]Dithiobis(Succinimidyl Propionate). Journal of Molecular Biology 1976, 104(1), 243-261. https://doi.org/10.1016/0022-2836(76)90011-5.
[4] Li, T.; Takeoka, S. Enhanced Cellular Uptake of Maleimide-Modified Liposomes via Thiol-Mediated Transport. Int. J. Nanomed. 2014, 9(1), 2849-2861. https://doi.org/10.2147/IJN.S58540
[5] Che, J.; Okeke, C. I.; Hu, Z.-B.; Xu, J. DSPE-PEG: A Distinctive Component in Drug Delivery System. Curr. Pharm. Des. 2015, 21(12), 1598-1605.
[6] Wang, J.; Zhang, R.-Y.; Wang, Y.-C.; Chen, X.-Z.; Yin, X.-G.; Du, J.-J.; Lei, Z.; Xin, L.-M.; Gao, X.-F.; Liu, Z.; Guo, J. Polyfluorophenyl Ester-Terminated Homobifunctional Cross-Linkers for Protein Conjugation. Synlett 2017, 28(15), 1934-1938. https://doi.org/10.1055/s-0036-1590974.
[7] Partis, M. D.; Griffiths, D. G.; Roberts, G. C.; Beechey, R. B. Cross-Linking of Protein by ω-Maleimido Alkanoyl N-Hydroxysuccinimido Esters. J Protein Chem 1983, 2(3), 263-277. https://doi.org/10.1007/BF01025358.
[8] Quanta BioDesign, personal observations with TFP ester reactions, 2019.
[9] Belowich, M. E.; Stoddart, J. F. Dynamic Imine Chemistry. Chem. Soc. Rev. 2012, 41(6), 2003-2024. https://doi.org/10.1039/C2CS15305J.
[10] Wendeler, M.; Grinberg, L.; Wang, X.; Dawson, P. E.; Baca, M. Enhanced Catalysis of Oxime-Based Bioconjugations by Substituted Anilines. Bioconjugate Chem. 2014, 25(1), 93-101. https://doi.org/10.1021/bc400380f.
[11] Larsen, D.; Kietrys, A. M.; Clark, S. A.; Park, H. S.; Ekebergh, A.; Kool, E. T. Exceptionally Rapid Oxime and Hydrazone Formation Promoted by Catalytic Amine Buffers with Low Toxicity. Chem. Sci. 2018, 9(23), 5252-5259. https://doi.org/10.1039/C8SC01082J.
[12] Kölmel, D. K.; Kool, E. T. Oximes and Hydrazones in Bioconjugation: Mechanism and Catalysis. Chem. Rev. 2017, 117(15), 10358-10376. https://doi.org/10.1021/acs.chemrev.7b00090.
[13] Baxter, E. W.; Reitz, A. B. Reductive Aminations of Carbonyl Compounds with Borohydride and Borane Reducing Agents. In Organic Reactions; American Cancer Society, 2004; pp 1-714. https://doi.org/10.1002/0471264180.or059.01.
[14] Fondo, M.; Corredoira-Vázquez, J.; GarcíaDeibe, A. M.; Sanmartín-Matalobos, J. An Easy Approach to Obtain Alcohol-Amines by Reduction of Alcohol Functionalized Imines. Proceedings 2019, 9(1), 2. https://doi.org/10.3390/ecsoc-22-05697.
[15] Billman, J. H.; Diesing, A. C. Reduction of Schiff Bases with Sodium Borohydride. J. Org. Chem. 1957, 22(9), 1068-1070. https://doi.org/10.1021/jo01360a019.
[16] Hutchins, R. O.; Hutchins, M. K.; Crawley, M. L.; Mercado-Marin, E. V.; Sarpong, R. Sodium Cyanoborohydride. In Encyclopedia of Reagents for Organic Synthesis; American Cancer Society, 2016; pp 1-14. https://doi.org/10.1002/047084289X.rs059.pub3.
[17] Tadanier, J.; Hallas, R.; Martin, J. R.; Stanaszek, R. S. Observations Relevant to the Mechanism of the Reductive Aminations of Ketones with Sodium Cyanoborohydride and Ammonium Acetate. Tetrahedron 1981, 37(7), 1309-1316. https://doi.org/10.1016/S0040-4020(01)92446-9.
[18] Abdel-Magid, A. F.; Carson, K. G.; Harris, B. D.; Maryanoff, C. A.; Shah, R. D. Reductive Amination of Aldehydes and Ketones with Sodium Triacetoxyborohydride. Studies on Direct and Indirect Reductive Amination Procedures1. J. Org. Chem. 1996, 61 (11), 3849-3862. https://doi.org/10.1021/jo960057x.
[19] Gribble, G. W.; Abdel-Magid, A. F. Sodium Triacetoxyborohydride. In Encyclopedia of Reagents for Organic Synthesis; American Cancer Society, 2007. https://doi.org/10.1002/9780470842898.rs112.pub2.
[20] Kim, T. H.; Jiang, H. H.; Lee, S.; Youn, Y. S.; Park, C. W.; Byun, Y.; Chen, X.; Lee, K. C. Mono-PEGylated Dimeric Exendin-4 as High Receptor Binding and Long-Acting Conjugates for Type 2 Anti-Diabetes Therapeutics. Bioconjugate Chem. 2011, 22(4), 625-632. https://doi.org/10.1021/bc100404x.
[21] Scheer, J. M.; Sandoval, W.; Elliott, J. M.; Shao, L.; Luis, E.; Lewin-Koh, S.-C.; Schaefer, G.; Vandlen, R. Reorienting the Fab Domains of Trastuzumab Results in Potent HER2 Activators. PLOS ONE 2012, 7(12), e51817. https://doi.org/10.1371/journal.pone.0051817.
Stay in the Loop. Join Our Online Community
Products
Ordering
About Us
Application
Resources
©Vector Laboratories, Inc. 2025 All Rights Reserved.
To provide the best experiences, we use technologies like cookies to store and/or access device information. Consenting to these technologies will allow us to process data such as browsing behavior or unique IDs on this site. Not consenting or withdrawing consent, may adversely affect certain features and functions. Privacy Statement