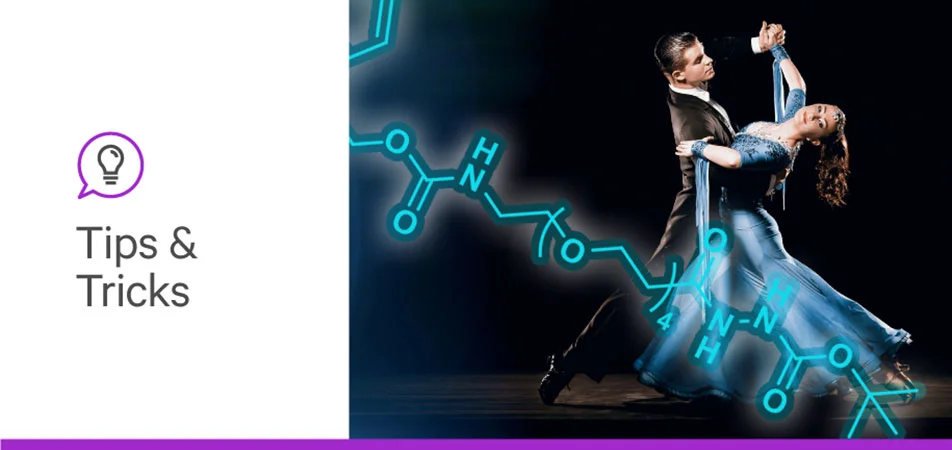
Vector Laboratories is closed for the President’s Day on Monday, February 19th. We will be back in the office on Tuesday, February 20th.
We will respond to emails upon our return. Have a wonderful day.
With knowledge of the applications that bioconjugation can play into, we finish by highlighting its key advantages and critical uses. While the applications are much broader than what we summarize here, we can deduce common threads between these methods.
Advantages of Bioconjugation
One of the highlights of bioconjugation is its contribution to targeting cells and tissues. In particular, the conjugation of proteins and peptides to nanoparticles, drugs, and imaging agents often helps to enhance the signal, minimize off-target interactions, which remains one of the most insurmountable bottlenecks in therapeutics and diagnostics, and label cells and tissues in vivo.
Efficient targeting is not the only advantage. In some cases, bioconjugation serves several other purposes to improve drug efficacy. For instance, drug molecules can be coupled with biomolecules to keep them inactive until they are taken up by the target host cells. Thus, they protect drugs from enzymatic degradation, extending their half-life and reducing the required dosage. Furthermore, the attachment of hydrophilic biomolecules can increase the solubility of the drug to enhance the circulation and absorption of injectable or orally administered drugs.
Perhaps the most important and overlooked aspect of bioconjugation is its biocompatibility. In other words, the involvement of biomolecules in functional enhancement ensures that the targeted therapeutic or diagnostic method is not cytotoxic to live and healthy cells. Therefore, bioconjugation provides an opportunity to visualize and track molecular interactions in live cells in real-time.
Challenges and Considerations in Bioconjugation
Despite the promising outcomes, bioconjugation methods can pose a few challenges, especially in the face of therapeutic protein manufacturing. That said, novel techniques are emerging to address these challenges and upgrade bioconjugation mechanisms.
Specificity is one of the main bottlenecks in successful bioconjugation. The components of a bioconjugate are coupled via a linkage group involving reactive functional groups of these molecules. For proteins, lysine and cysteine are reactive sites ideal for conjugation. However, their high relative abundance in a protein can lead to heterogeneity in the number and the location of conjugation sites and disrupt the native function of the biomolecule, which can lead to undesired effects or weakened efficacy in the body.
With the emergence of site-specific bioconjugation methods, researchers can gain a much higher level of control over the structure and function of protein conjugates. By ensuring that the covalent attachment only occurs at a specific site, they can prevent unprecedented structural alterations that might disrupt the function of the biomolecule and trigger immunogenicity.
Furthermore, site-specific conjugation makes fine-tuning much easier when investigating the optimum stoichiometry and ligand-to-target ratio [11].
The concept of multivalency is another exciting upgrade in bioconjugation, where a central molecule can be attached to multiple copies of a biomolecule, resulting in enhanced target affinity and enhanced therapeutic effect. However, the technique brings on additional problems in synthesis, such as stoichiometric control, the risk of steric hindrance, and difficulty of intracellular delivery due to increased size. Furthermore, tracking the structural integrity and detecting batch-to-batch variability can be challenging, which hinders their regulatory approval and translation into clinical settings.
Future Directions
Bioconjugation technologies continue to evolve thanks to novel chemical, enzymatic, genomic, and computational methods. Among the most influential upgrades are click chemistry methods for improving selectivity and maximizing yield and bioorthogonal methods enabling bioconjugation of molecules in living cells. With the involvement of genomics, automation, and computational science, more improvements are on the horizon.
As mentioned in the previous section, site-specific labeling has been a pain point in bioconjugation. Genetically encoded bioconjugation overcomes this through genetic modification of the protein to give rise to a predetermined bioconjugation site. The incorporation of unnatural amino acids into the gene sequence gives rise to a modified protein containing a functional group designed for bioconjugation [12].
Additionally, high-throughput screening methods can be implemented for identifying favorable conjugation sites. For example, Yamazoe et al. used mass spectrometry to analyze antibody-drug conjugate libraries to rapidly discover novel conjugation sites [13].
Another group of advancements aims to facilitate better stoichiometric control and integrate bioconjugation in high-throughput settings. For example, photo-click chemistry makes use of UV or visible light to trigger covalent bonds, allowing spatiotemporal control of bioconjugation reactions. Photoreactive groups are crucial to controlling the timing and site of covalent bond formation [14].
Recently, microfluidic devices have been employed for precise and controlled bioconjugation. Microfluidic chips contain networks of channels and chambers that help control reaction volumes as well as temperature and pH. The precision bestowed by microfluidics greatly enhances reproducibility and can aid high-throughput bioconjugation.
The advancements in stem cell research have positive implications for bioconjugation as well. 3D models derived from stem cells, such as organoids, are much better at recapitulating the physicochemical properties of tissues. Thus, using bioorthogonal conjugation methods in these models can help researchers gather biologically relevant data regarding structure and function.
Conclusion
With a series of three blog posts, we introduce Bioconjugation, which is a powerful and versatile technique that can merge a biomolecule with various types of small molecules to unlock functions that neither standalone component could exhibit. It can give biochemical modalities that can serve as promising tools in targeted therapies and diagnostics and can be integrated with nanotechnology. It is not without trials and tribulations; successful linkage between two molecules requires meticulous experimental procedures for selective and homogenous conjugation. However, constant improvements in click chemistry methods and cutting-edge analytical platforms will support biochemists in their quest to fulfill the criteria. Detailed explanations of our bioconjugation products can be found in the Vector Laboratories Bioconjugation Resource Guide and Bioconjugation Application Page. To learn more about bioconjugation and other ways to advance your scientific research, stay tuned!
1. Algar, W.R., A brief introduction to traditional bioconjugate chemistry. Chemoselective and bioorthogonal ligation reactions: concepts and applications, 2017. 1: p. 1-36.
2. Mahesh, S., K.-C. Tang, and M. Raj, Amide bond activation of biological molecules. Molecules, 2018. 23(10): p. 2615.
3. Wenner, W., Phenylacetamide. Org Synth, 1952. 4: p. 760.
4. Stenzel, M.H., Bioconjugation using thiols: old chemistry rediscovered to connect polymers with nature’s building blocks. 2013, ACS Publications.
5. Presolski, S.I., V.P. Hong, and M. Finn, Copper‐catalyzed azide–alkyne click chemistry for bioconjugation. Current protocols in chemical biology, 2011. 3(4): p. 153-162.
6. Brassard, J., et al., Antibody-drug conjugates targeting tumor-specific mucin glycoepitopes. Frontiers in Bioscience-Landmark, 2022. 27(11): p. 301.
7. Parakh, S., et al., Radiolabeled antibodies for cancer imaging and therapy. Cancers, 2022. 14(6): p. 1454.
8. Shevtsov, M.A., et al., Superparamagnetic iron oxide nanoparticles conjugated with epidermal growth factor (SPION–EGF) for targeting brain tumors. International journal of nanomedicine, 2014: p. 273-287.
9. Huang, Y., et al., Superparamagnetic iron oxide nanoparticles conjugated with folic acid for dual target-specific drug delivery and MRI in cancer theranostics. Materials Science and Engineering: C, 2017. 70: p. 763-771.
10. Ren, X., X. Meng, and F. Tang, Preparation of Ag–Au nanoparticle and its application to glucose biosensor. Sensors and Actuators B: Chemical, 2005. 110(2): p. 358-363.
11. Lieser, R.M., et al., Site-specific bioconjugation approaches for enhanced delivery of protein therapeutics and protein drug carriers. Bioconjugate chemistry, 2020. 31(10): p. 2272-2282.
12. Travis, C.R., et al., Genetic encoding of a bioconjugation handle for [2+ 2+ 2] cycloaddition reactions. ChemBioChem, 2020. 21(3): p. 310-314.
13. Yamazoe, S., et al., High-Throughput Platform to Identify Antibody Conjugation Sites from Antibody–Drug Conjugate Libraries. Bioconjugate Chemistry, 2020. 31(4): p. 1199-1208.
14. Wan, C., et al., The thiol-sulfoxonium ylide photo-click reaction for bioconjugation. Chemical Science, 2023. 14(3): p. 604-612.
15. Lee, S., et al. (2014). Labeling and Tracking Method without Distorted Signals by Phagocytosis of Macrophages. Theranostics, 4 (4), 420-31.
16. Murrey, H. E, et al. (2015). Systematic Evaluation of Bioorthogonal Reactions in Live Cells with Clickable HaloTag Ligands: Implications for Intracellular Imaging. J Am Chem Soc., 137 (35), 11461-75.
17. Kim, K., et al. (2016). Bioorthogonal Copper Free Click Chemistry for Labeling and Tracking of Chondrocytes In Vivo. Bioconjug Chem., 27 (4), 927-36.
Stay in the Loop. Join Our Online Community
Together we breakthroughTM
©Vector Laboratories, Inc. 2024 All Rights Reserved.