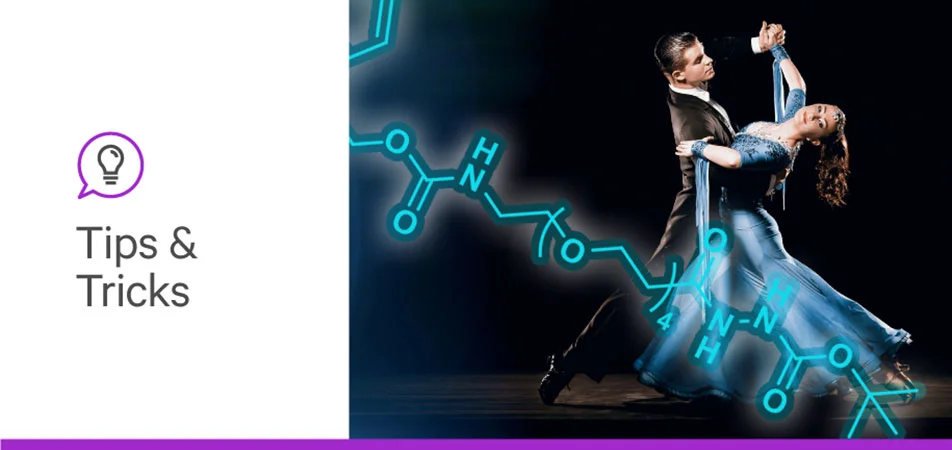
Vector Laboratories is closed for the President’s Day on Monday, February 19th. We will be back in the office on Tuesday, February 20th.
We will respond to emails upon our return. Have a wonderful day.
The traditional approach to disease biology typically involves collecting causes, symptoms, and treatments under one umbrella. Current therapeutics are designed to target the hallmarks of a disease, such as an undesired protein-protein interaction or excessive activity of an enzyme.
Target information is often extracted from a set of biomarkers, measurable indications of the disease state. However, the patient profile is too diverse to do this for many diseases. Especially in cancer and neurodegenerative diseases, we cannot underestimate the number of patients who do not respond to traditional small-molecule drug treatments.
The failure of current small-molecule drug treatments in patients points to the need for an adequate biomarker portfolio. We need to dig deeper to find more distinct biomarkers for detailed diagnosis, prediction of treatment outcomes, and the implementation of personalized approaches, such as immunotherapy. In particular, post-translational modifications (PTMs) must be prioritized due to their roles in diversifying the functional space of proteins.
Glycosylation is one of the most common and perplexing PTMs. The glycosylation of a macromolecule determines its fate in many aspects, from its subcellular localization to its potential binding partners. Glycosylation can occur in numerous combinations, giving rise to a diverse glycan profile, which could greatly vary between healthy and diseased states. This means that differential glycan profiles can be analyzed to identify disease states more accurately.
Here, we give examples of how glycans can be exploited as robust biomarkers.
As we previously discussed in our blog post, “An Introduction to the Universe of Glycans”, glycans are classified according to the biomolecule they are attached to. All 3 classes—glycoproteins, proteoglycans, and glycolipids, contain strong indicators of disease.
Many FDA-approved protein-based cancer biomarkers are glycoproteins, including the CA 125 antigen for ovarian cancer, CA 19-9 for pancreatic cancer, and CA 15-3 for breast cancer (1–3). Studying the glycosylation trend of these antigens can improve their diagnostic value in cancer.
Heavily-glycosylated proteoglycans can be particularly valuable as metastatic cancer biomarkers. Circulating tumor cells (CTCs) are prevalent in liquid biopsies, and their metastatic properties are bestowed by proteoglycans (4). Furthermore, the sulfation of the glycosaminoglycan chains varies across different cancer types, triggering metastatic events such as angiogenesis, epithelial-to-mesenchymal transition, and movement across the blood vessel wall (5).
Glycosphingolipids, particularly gangliosides, emerged as biomarkers for brain pathologies since they constitute 80% of the glycan mass in the brain (6). Changes in ganglioside levels are strongly associated with many neurodegenerative diseases, including epilepsy, stroke, multiple sclerosis (MS), Parkinson’s disease (PD), Huntington’s disease, and Alzheimer’s disease (AD) (7).
A growing body of research reveals the bidirectional relationship between glycosylation patterns and cancerous behavior. Altered glycosyltransferase expression changes the surface glycan composition and triggers signaling cascades that transform cells into malignant phenotypes. Such cells contain an increased abundance of cancer-associated glycan motifs, such as truncated O-glycans, branched N-glycans, sialyl lewis antigens, and core fucosylation (8).
In addition, cancer cells can modulate their glycosylation patterns depending on the conditions of their tumor microenvironment. The most obvious example is the changes in glycans as cancer progresses from an initial stage—with uncontrolled proliferation—to an advanced stage where metastasis occurs due to hypoxic conditions. The cells can adapt by attaining a distinct glycan profile to prepare for metastasis and to protect themselves against immune response and insufficient oxygen supply.
In other words, glycans are valuable assets for biomarker studies to not only discern between healthy and cancerous cells but also determine disease prognosis and subtype. The expansion in the cancer biomarker portfolio can drive accurate and timely diagnosis of cancer types and stages.
Among them, prostate cancer presents particular diagnostic challenges due to the weak specificity of conventional prostate-specific antigens (PSAs). Efforts to identify and measure glycans attached to the PSA can improve the accuracy of detection tools. For example, researchers from the Hirosaki University Graduate School of Medicine in Japan designed an immunoassay system to differentiate between benign and malignant cell types in the prostate.
They successfully differentiated between α2,3-linked sialyl N-glycan-carrying PSA and benign-associated α2,6-linked sialyl N-glycan-carrying PSA using the plant lectin Maackia amurensis, which specifically binds the former (9).
Single glycan biomarkers are insufficient to encompass the complexity of the multiple reactions participating in cancer phenotypes. Current research emphasizes glycoprotein panels through liquid chromatography-mass spectrometry (LC-MS/MS) to simultaneously identify and quantify multiple glycoproteins. Thanks to the advancements in LC-MS/MS methods, researchers can even detect cancer-associated glycopeptides and proteins in very low relative abundance (10).
The role of glycosylation in the central nervous system (CNS) came to light as scientists could investigate the neural proteome more elaborately. Today, studies uncover strong associations between glycogenes and Alzheimer’s disease, multiple sclerosis, Parkinson’s disease, and Huntington’s disease.
Studies implicate P-glycoprotein as a potential biomarker and target in Alzheimer’s and Huntington’s disease, where the overabundance of the P-glycoprotein was strongly correlated with the accumulation of amyloid-β peptide and mutant huntingtin protein, respectively (11,12).
Multiple sclerosis research focuses on myelin oligodendrocyte glycoprotein (MOG) and its role in disease progression. For example, Khare et al. showed that MS-associated antibodies induced autoimmune brain inflammation by binding MOG (13). Furthermore, MOG was shown to stimulate inflammatory cytokine production by T-cells (14).
Multiple glycoproteins are at play in Parkinson’s disease. Dopaminergic dysfunction and neuronal degeneration have been linked to synaptic vesicle glycoprotein 2, the transmembrane glycoprotein GPNMB, and myelin-associated glycoprotein (15–17).
In summary, identifying and quantifying the above glycoproteins—and many others—in the CNS can generate valuable insights into the onset of neurodegenerative systems. For example, one study employed biotinylated Agaricus bisporus lectin to detect the Gal-(beta-1,3)-GalNAc motif in microtubule-associated protein (MAP6). They unveiled hyperglycosylation of MAP6 in PD mouse models compared to the control model, which affirms the potential of MAP6 glycosylation as a robust PD biomarker (18).
Life expectancy has drastically increased since the 20th century. This caused the emergence of age-related diseases as the human body inevitably deteriorates and long-term effects of harmful habits like alcohol and processed food consumption manifest over time.
Age is a risk factor for cancer and neurodegenerative diseases, but other age-related diseases and chronological aging can involve similar mechanisms in glycosylation.
The N-glycome was shown to be particularly sensitive to chronological aging. Analysis of serum proteins revealed a general increase in agalactosyl and non-sialylated N-glycans and a decrease in core-fucosylated biantennary N-glycans (19). Furthermore, the course of glycan changes was subtle before 50 and more rapid afterwards (20). These results prove galactosylation and sialylation to be viable indicators of age-related physiological changes.
In addition, the ratio of 2 glycans can also predict and inform age-related health decline. To that end, researchers have developed an age-related biomarker named GlycoAgeTest that measures the ratio between 2 fucosylated biantennary oligosaccharides (N2GAF:NA2F). The test revealed a gradual increase in the ratio after 40, with implications for dementia (21).
Differential glycosylation has also been demonstrated in Type 2 diabetes mellitus, metabolic syndrome, cardiovascular diseases, and chronic inflammation (22). Therefore, timely detection of glycan alterations is necessary, as it can help us take preventative measures against the debilitating effects of age-related conditions.
We need to employ several complementary methods to establish glycans as efficient biomarkers. These methods exploit the specificity of glycan-binding agents, such as lectins and antibodies, to deduce the diagnostic value of various glycans.
Flow cytometry and mass spectrometry can be used to analyze glycan content in liquid biopsies using glycan binders (23,24). On the other hand, genome-wide glycan profiling requires high-throughput methods, such as glycan arrays, which can identify several glycans with their binding partners and quantify binding using fluorescence-based detection (25).
Quantitative methods can be complemented with immunohistochemistry and immunofluorescence that help visualize the subcellular glycan distribution and impact on cell phenotype. Lastly, capillary electrophoresis augments specificity by allowing the separation of isomers—glycans harboring the same monosaccharide sequence but different conformations (26).
The strong correlation between disease and altered glycan profile is evident. More importantly, detailed glycan profiling reveals a heterogeneous patient profile in many diseases, especially cancer. Current research must address some of the hurdles in glycan discovery, such as detecting previously-unknown complex glycan sequences, differentiating between benign and malignant types, and elucidating the downstream effects of aberrant glycosylation.
Nevertheless, researchers continue to generate novel insights thanks to the expanding knowledge of glycan binders and the combination of analytical techniques. Every milestone in this area will contribute to the early detection and detailed profiling of cancer subtypes, which is necessary for predicting the best treatment option for every patient.
Comprehensive glycobiology expertise is a must to achieve milestones in glycan biomarker discovery. That’s why we offer not only a large selection of lectins as detection kits but also educational resources. A recent article, “Leveraging Glycobiology for Biomarker Discovery”, from Pamela James, Vice President, Product at Vector Laboratories offers a concise outlook on glycobiology and its importance in drug discovery. In addition, you can refer to the Intro to Glycobiology ebook and SpeakEasy Science blog to get a better idea of how we can assist with your research questions.
Stay in the Loop. Join Our Online Community
Together we breakthroughTM
©Vector Laboratories, Inc. 2024 All Rights Reserved.